Applying biochar to soil has been shown to improve carbon sequestration and soil health, and remediate contaminated soil and water resources. Biochar is valuable as a nutrient source and a soil amendment, to remediate contaminated soil and aquatic ecosystems. In this article, we present an overview on the synthesis and characteristics of biochar, and how designer biochar may help remediate contaminated soil and water.
Biochar synthesis
Biochar is charcoal made thermochemically from biomass. The starting material can be various carbon-based sources, including woody biomass, crop residues, animal litter, composts and biosolids. Thermochemical processes used include pyrolysis, torrefaction and hydrothermal carbonisation.
Pyrolysis
Pyrolysis is an inexpensive and robust technology, which can thermochemically decompose organic matter into non-condensable syngases, condensable bio-oils and solid residual products – biochar. The end product of pyrolysis can be controlled by altering the pyrolysis parameters, such as temperature and length of the process. For example, increasing the pyrolysis temperature increases gas yield and decreases biochar production.
Torrefaction
Torrefaction is a modified pyrolysis technique. Here, biomass is slowly heated within a specified temperature range (200–300 °C) and held for a certain duration to remove the moisture, carbon dioxide and oxygen from feedstocks (raw materials). The torrefaction environment must be inert or have limited oxygen. Torrefaction temperature is the most important parameter that influences the biomass weight loss, and the chemical and thermophysical properties of biochar. The torrefied biomass has properties similar to that of raw biomass and biochar, because torrefaction is first stage of the pyrolysis process and the torrefied biomass still contains some volatile organic compounds.
Hydrothermal carbonisation
Hydrothermal carbonisation (HTC) is an exothermic process in which feedstock is heated in water in a confined system. HTC offers advantages for biochar production, including high-conversion efficiency and relatively low operating temperature (150–250 °C), and it does not use an energy-extensive drying process. The composition and structure of the biochar (hydrochar) from HTC differs substantially from that of pyrolysis biochar. The chemical structure of hydrochar closely resembles natural coal, with higher hydrogen:carbon (H:C) and oxygen:carbon (O:C) ratios than biochar from pyrolysis.
Characteristics of biochar
Physical characteristics
Physical characteristics, such as surface area and porosity of biochar, are key parameters controlling the magnitude of interactions between biochar and contaminants. The physical and chemical properties of biochar are affected by several factors, including:
- feedstock types
- production conditions such as pyrolysis temperature
- gas flow
- presence of gases, such as carbon dioxide or nitrogen.
For example, higher temperatures during biochar synthesis result in the biochar-pore infillings releasing volatile matter. This produces biochar with maximum surface area and microporosity. Resulting pore sizes differ, and the relative distribution of pore size affects interactions between biochar and the environmental solutes, including contaminants.
Elemental characteristics
Biochar is made up of elements such as carbon, hydrogen, sulfur, potassium, oxygen and nitrogen, as well as minerals in its ash component. The elemental composition of biochar also depends on its production conditions and feedstock types. Elemental molar ratios, such as H:C and O:C, of biochar are used to measure its aromaticity and maturation. Aromaticity is defined as the fraction of carbon in biochar that forms aromatic bands. Biochar with dense aromatic structures is more resistant to oxidation and microbial degradation. Therefore, the degree of aromatic condensation in biochar is related to its recalcitrance in the environment. Low-temperature biochar is likely to have lower aromaticity than high-temperature biochar.
pH and Eh of biochar
Biochar is typically alkaline, but this depends on the original feedstock and the preparation conditions. A higher ash content results in a biochar with a higher pH. Functional groups, such as carboxylic and phenolic groups, in biochar determine its surface charge and redox properties, and play an important role in the retention of cations and anions in soil and water. Biochar can donate, accept or transfer electrons to or from their surrounding environments, thereby influencing the redox reactions of contaminants.
Elemental composition, cation-exchange capacity, surface functional groups, and pH and Eh of biochar are important properties that control the interactions between biochar and contaminants.
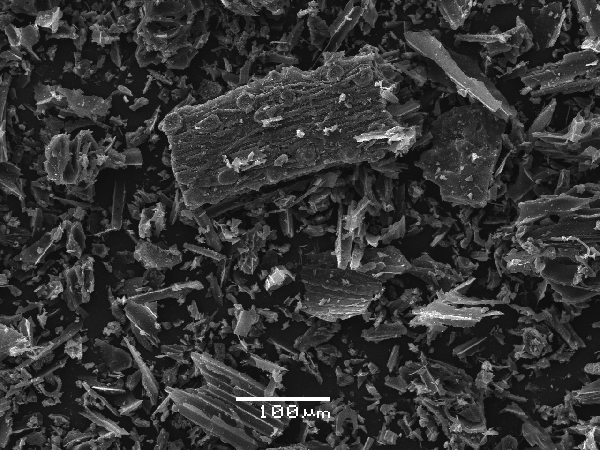
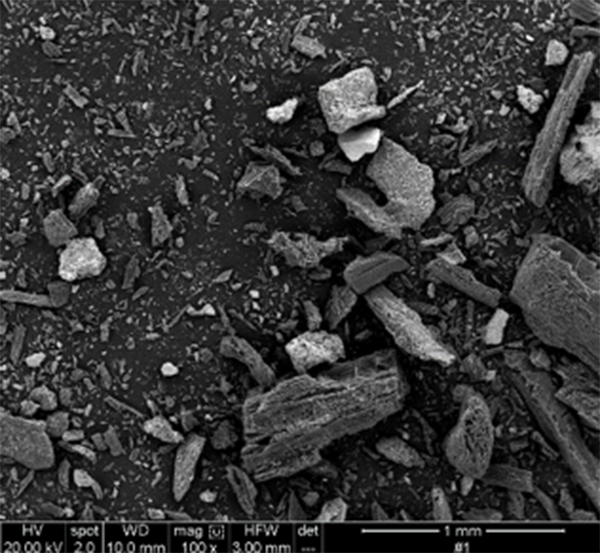
Making designer biochar
Recently, the concept of designing biochar for a specific purpose has been proposed. Using designer biochar is reported to be more effective than simply applying biochar indiscriminately. For example, modifying the biochar surface aims to improve the physicochemical and sorptive characteristics of biochar for novel uses such as heavy metal binding, organic pollutant sorption and water purification.
Various approaches are used to modify biochar surface properties. Here, we discuss impregnation, oxidation and grafting.
Impregnation
Impregnation is a process where elements (usually metals) are incorporated into the biochar surface through prolonged wet contact. Biochar modification by impregnation is likely to make them more active in terms of retaining heavy metals from aqueous solution. Nitric acid is often used to remove intrinsic ash and tar from biochar before impregnation.
Oxidation
Oxidation is another modification process where liquid or gaseous compounds (eg inorganic acids) are used to modify and purify biochar. Two types of oxidation, liquid-phase oxidation and gas phase oxidation, are generally used.
In the liquid-phase oxidation process, the following have been used to modify or purify biochar:
- strong acids such as nitric acid
- a mixture of nitric and sulfuric acids
- strong oxidisers, such as potassium permanganate, in an acid solution
- other chemical mixtures, such as hydrogen peroxide/sulfuric acid and hypochlorite solution.
Gaseous phase oxidation uses carbon dioxide and oxygen gas to modify the biochar. Using potassium hydroxide increases the biochar’s porosity and surface area.
Oxidation can modify biochars in 2 different ways:
- it opens the pores in char products and then fills the pores with metals
- it adds new functional groups on the surface of biochars.
Acid and base chemical activation modifies biochar through both dehydration and oxidation. The feedstock is usually mixed or soaked in a solution of acids and bases before pyrolysis and activation. Strong oxidisers also can be used to modify or purify biochar.
Grafting
Grafting can also modify biochar, by fusing guest molecules or elements onto the functional groups of the char surface, using the condensation reaction. Grafting can be done with or without pre-oxidation. There are several compounds or materials that can be used to graft biochar. Grafted biochar has many potential applications, such as in biomaterials, bioengineering and emerging biotechnology.
Biochar-assisted remediation of heavy metals
Biochar application is effective in the remediation of heavy metal–contaminated soil and aquatic ecosystems. Various mechanisms have been proposed to explain how biochar remediates heavy metal contamination, but chemosorption is the dominant process involved in removing heavy metals such as cadmium and lead in soil and aquatic systems.
Biochar-mediated redox reactions controlling the (im)mobilisation and subsequent bioavailability of heavy metals have been reported. For example, although chromium(III) is strongly retained onto soil particles, Cr(VI) is very weakly adsorbed and is readily available for plant uptake and leaching to groundwater. Thus, reduction of Cr(VI) to Cr(III) can affect the (im)mobilisation and bioavailability of chromium. Although oxidation of Cr(III) to Cr(VI) is mostly mediated abiotically through oxidising agents – such as manganese oxide and, to a lesser extent, ferric oxide – reduction of Cr(VI) to Cr(III) is mediated through abiotic and biotic processes. Chromate can be reduced to Cr(III) in environments that have electrons available. Biochar provides a source of electrons, thereby enhancing the reduction of Cr(VI) to Cr(III) and subsequent immobilization of Cr(III) – mitigating the environmental toxicity of chromium.
Conclusions
Applying biochar to soil has been shown to improve carbon sequestration, soil health and agricultural productivity. Currently, the focus of biochar research is on its potential value in mitigating the toxicity of contaminants in soil and groundwater resources. Its favourable physicochemical characteristics – such as high surface area, porosity and surface charge density of biochar – are key parameters enabling its potential application to remediate contaminated soil and aquatic ecosystems.